Finite Element Modeling of the Lamina Cribrosa of the Optic Nerve Head in Glaucoma
The FE models shown in this report were constructed and analyzed by the Ocular Biomechanics Laboratory, led by Dr. J. Crawford Downs, using 3D histomorphometric data from the Optic Nerve Head Research Laboratory, directed by Dr. Claude Burgoyne. Both laboratories are part of the Devers Eye Institute at Legacy Health System, which is based in Portland, Oregon, USA. This work is funded by the National Institutes of Health grant EY011610.
|
|
Glaucoma is a disease of the eye that gradually and relentlessly narrows the field of vision. If left untreated, this progression of damage can culminate in total blindness. Glaucoma is the second leading cause of blindness worldwide, and despite extensive and prolonged research efforts, the mechanisms that initiate and fuel progression of the disease are not well understood. It is known, however, that interventions to reduce the pressure load within the eye – lowering intraocular pressure (IOP) – are the only therapies clinically proven to be effective in slowing or halting glaucomatous progression. Still, the relationship between IOP and glaucoma is controversial because not all patients with elevated IOP develop glaucoma, nor are all patients with low or normal IOP immune to the disease. Thus, there is a population of "susceptible" patients who must be identified and treated at the earliest possible time in the disease process to minimize non-recoverable vision loss.
The prevailing evidence implicates a specific region in the back of the eye, the optic nerve head, as the site of glaucomatous damage. This is the region where the long axonal processes of the cells of the retina converge and pass through the wall of the corneo-scleral shell to form the optic nerve, the structure that conveys visual information to the brain. Within the optic nerve head there is a load-bearing fenestrated connective tissue network called the lamina cribrosa (LC) that spans the opening in the corneo-scleral envelope through which the axon bundles pass. This porous network of connective tissue beams is vascularized (i.e., most individual, load-bearing laminar beams enclose a capillary) with astrocytic cells residing on the surfaces of the beams to help maintain the integrity of their collagenous extracellular matrix and support the metabolic needs of the adjacent axon bundles.
One of the aims of our research program is to characterize the mechanical environment of the connective tissues of the posterior scleral shell and LC at the macro- and micro scales. Through these efforts, we hope to relate the global effect of IOP variation to the local mechanical environment of the connective tissue beams in the LC, and eventually relate these quantities to subsequent changes in tissue composition, cellular proliferation and activity, and capillary perfusion and blood flow.
To address the role of biomechanics in the development of glaucoma, our laboratories have developed a technique to serially reconstruct the complex connective tissue microarchitecture of the LC using a microtome-based block face imaging method. An example of such a rendered 3D reconstruction is shown in Figure 1. The geometries from these 3D reconstructions form the basis of our multi-scale finite element analyses of the posterior eye and LC.
We utilize MSC/PATRAN in the construction of our finite element models and for visualization of finite element analysis results. The geometry and meshing tools available in PATRAN allow us to construct models which respect the anatomic fidelity of individual-specific eye models (e.g., thickness variations in different regions of the eye) and the post-processing tools help us to critically inspect results to help guide our research efforts. We also harness the power of the PCL programming language to automate various model building tasks which routinely occur in our data pipeline.
As shown in Figure 1, the microarchitecture of the LC is extremely complex and inhomogeneous. To account for this complexity within our FE models, we have adopted a continuum-based approach to model the posterior pole of the eye such that assignment of material properties within the LC elements is based on local microarchitectural information from the 3D reconstruction (Figure 2). The displacement results from this continuum analysis (Figure 3) are then used to apply displacement boundary conditions to detailed microFE models of subregions of the LC (Figure 4). When these microFE models are analyzed, the resultant stresses and strains at the beam level exhibit a great deal of spatial complexity (Figure 5). We hope to utilize this multi-scale FEA approach to correlate characteristics of load transmission and strain within the LC with experimentally and histologically measured indicators of glaucomatous progression.

Figure 1. An anatomic geometry of the posterior half of the eye with a close up view of the optic nerve head and surrounding structures. Within the optic nerve head, there is a porous, load-bearing connective tissue called the lamina cribrosa (LC) that spans the scleral canal. Axon bundles from the retina converge at the optic nerve head, turn, and pass through the LC on their way to the brain. The LC is believed to be the primary site of axonal damage in glaucoma, but the specific mechanism of insult is unclear. We are using biomechanical engineering approaches to characterize the mechanical environment within the connective tissues of the LC to better understand how load bearing at this site might relate to the cascade of injury leading to glaucomatous vision loss.
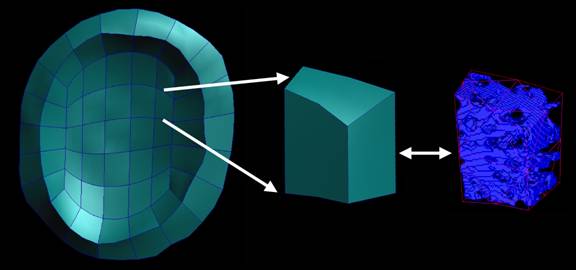
Figure 2. The portion of the posterior pole continuum finite element model corresponding to the optic nerve head is aligned with the 3D voxel-based reconstruction of the LC so that the specific tissue microarchitecture can be associated with each finite element. Orthotropic material properties for each parent LC continuum element are calculated from the enclosed LC tissue microarchitecture using a fabric tensor-based approach.

Figure 3. A finite element model of the posterior pole of the eye is constructed with microarchitecture-dependent orthotropic material properties assigned to the region corresponding to the lamina cribrosa. Here, we show the displacement field within the LC elements due to an IOP elevation of 30mmHg. Note that the nonuniform thickness of model and the nonuniform material property assignment within the lamina cribrosa produces an asymmetric displacement in the region of interest.
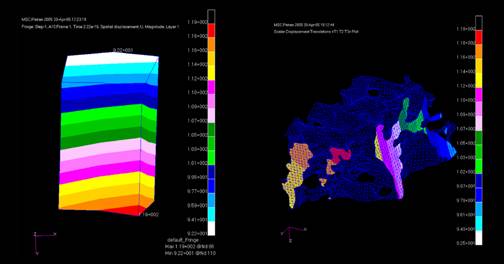
Figure 4. A 20-noded hexahedral continuum element from the LC region of the continuum level model shows the displacement field within that particular element (left). We use the shape functions of the 20-node hexahedral elements to transfer the solved displacements from a homogenized continuum level analysis onto a detailed microFE model (right) where they serve as boundary conditions for a subsequent analysis. Note that the microFE model has been rotated to show the applied displacements on two faces corresponding to the right and bottom faces of the parent continuum element.

Figure 5. The applied displacement boundary conditions (right) lead to the microFE solutions shown here. On the left, the displacement field across the microFE model mimics the continuum level solution for the parent element (left). On the right, the von Mises stress within the microFE model shows the complexity of the mechanical environment to which the connective tissue and resident cell populations are exposed.
No comments:
Post a Comment